Теоретичне обґрунтування можливості використання корене-поліноміальних функцій різного порядку для інтерполяції та апроксимації граничної траєкторії електронного пучка
DOI:
https://doi.org/10.20535/S0021347023020024Ключові слова:
електронна гармата, високовольтний тліючий розряд, транспортування електронних пучків, просторовий заряд, компенсація просторового заряду, залишковий газ, функціональний аналіз, інтерполяція, апроксимація, корене-поліноміальна функціяАнотація
У статті, з використанням методів функціонального аналізу, теоретично обґрунтована можливість застосування корене-поліноміальних функцій різних порядків для інтерполяції та апроксимації граничної траєкторії електронного пучка у разі його поширення в іонізованому газі з компенсацією просторового заряду електронів пучка. Показано, що саме корене-поліноміальні функції задовольняють диференціальному рівнянню другого порядку, яке описує граничну траєкторію електронів пучка за таких фізичних умов. Наведені результати інтерполяції та апроксимації граничної траєкторії електронного пучка корене-поліноміальними функціями з другого до п’ятого порядку. Результати інтерполяції порівнюються з результатами розв’язку диференціального рівняння для граничної траєкторії електронного пучка числовим методом Рунге–Кутта четвертого порядку, які для завдання інтерполяції вважаються еталонними. Для розв’язування завдання апроксимації в статті запропоновано ітераційний алгоритм, оснований на обчисленні у відлікових точках як значень функції, так і її похідних. Завдання апроксимації розв’язане для вибірки числових даних, отриманих на експериментальному електронно-променевому обладнанні для реальних процесів сучасних електронно-променевих технологій, що обумовило досить велике значення експериментальної похибки вимірювання через дію випадкових факторів, пов’язаних з термічною обробкою виробів електронним променем. Тестові розрахунки показали, що похибка інтерполяції та інтерполяції числових даних, які описують граничну траєкторію електронного пучка у разі його поширення в іонізованому газі, не перевищує кількох відсотків.
Посилання
[1]I. Melnyk, S. Tyhai, and A. Pochynok, “Universal Complex Model for Estimation the Beam Current Density of High Voltage Glow Discharge Electron Guns”, in Advances in Information and Communication Technology and Systems, Cham: Springer International Publishing, 2020, pp. 319–341.
[1]S. Denbnovetsky, “Principles of operation of high voltage glow discharge electron guns and some possibilities of their technological application”, in Photonics Applications in Astronomy, Communications, Industry, and High-Energy Physics Experiments 2017, Wilga, Poland, 2017, p. 104455R.
[1]S. Denbnovetsky, V. Melnyk, and I. Melnyk, “High-voltage, glow-discharge electron sources and possibilities of its application in industry for realizing different technological operations”, IEEE Transactions on Plasma Science, vol. 31, no. 5, pp. 987–993, Oct. 2003.
[1]S. V. Denbnovetsky, “Model of control of glow discharge electron gun current for microelectronics production applications”, in Sixth International Conference on Material Science and Material Properties for Infrared Optoelectronics, Kiev, Ukraine, 2003, p. 64.
[1]И. В. Мельник, “Оценка времени увеличения тока высоковольтного тлеющего разряда в триодной электродной системе при подаче управляющих импульсов”, Известия высших учебных заведений. Радиоэлектроника, vol. 56, no. 12, pp. 51–61, Dec. 2013.
[1]I. Khytruk, A. Druzhinin, I. Ostrovskii, Y. Khoverko, N. Liakh-Kaguy, and K. Rogacki, “Properties of Doped GaSb Whiskers at Low Temperatures”, Nanoscale Research Letters, vol. 12, no. 1, Feb. 2017.
[1]A. Druzhinin, I. Bolshakova, I. Ostrovskii, Y. Khoverko, and N. Liakh-Kaguy, “Low temperature magnetoresistance of InSb whiskers”, Materials Science in Semiconductor Processing, vol. 40, pp. 550–555, Dec. 2015.
[1]A. Zakharov, S. Rozenko, S. Litvintsev, and M. Ilchenko, “Trisection Bandpass Filter With Mixed Cross-Coupling and Different Paths for Signal Propagation”, IEEE Microwave and Wireless Components Letters, vol. 30, no. 1, pp. 12–15, Jan. 2020.
[1]A. Zakharov, S. Litvintsev, and M. Ilchenko, “Trisection Bandpass Filters With All Mixed Couplings”, IEEE Microwave and Wireless Components Letters, vol. 29, no. 9, pp. 592–594, Sep. 2019.
[1]A. Zakharov, S. Rozenko, and M. Ilchenko, “Varactor-Tuned Microstrip Bandpass Filter With Loop Hairpin and Combline Resonators”, IEEE Transactions on Circuits and Systems II: Express Briefs, vol. 66, no. 6, pp. 953–957, Jun. 2019.
[1]A. Zakharov, S. Litvintsev, and M. Ilchenko, “Transmission Line Tunable Resonators With Intersecting Resonance Regions”, IEEE Transactions on Circuits and Systems II: Express Briefs, vol. 67, no. 4, pp. 660–664, Apr. 2020.
M. I. Grechanyuk, A. G. Melnyk, I. M. Grechanyuk, V. G. Melnyk, D. V. Kovalchuk, “Modern electron beam technologies and equipment for melting of metals and alloys, deposition of protective coatings, production of composites condensed from vapor phase and powders,” Electrotech. Electron., vol. 49, no. 5–6, pp. 115–121, 2014, uri: https://epluse.ceec.bg/modern-electron-beam-technologies-and-equipment-for-melting-of-metals-and-alloys-deposition-of-protective-coatings-production-of-composites-condensed-from-vapor-phase-and-powders/.
G. Mattausch et al., “Gas discharge electron sources – proven and novel tools for thin-film technologies,” Electrotech. Electron., vol. 49, no. 5–6, pp. 183–195, 2014.
V. Vassilieva, K. Vutova, D. V., “Recycling of alloy steel by electron beam melting,” Electrotech. Electron., vol. 47, no. 5–6, pp. 142–145, 2012.
[1]H. Sasaki, Y. Kobashi, T. Nagai, and M. Maeda, “Application of Electron Beam Melting to the Removal of Phosphorus from Silicon: Toward Production of Solar-Grade Silicon by Metallurgical Processes”, Advances in Materials Science and Engineering, vol. 2013, pp. 1–8, Jan. 2013.
[1]T. Kemmotsu, T. Nagai, and M. Maeda, “Removal Rate of Phosphorus from Molten Silicon”, High Temperature Materials and Processes, vol. 30, no. 1-2, Jan. 2011.
[1]J. Pires, J. Otubo, A. Braga, and P. Mei, “The purification of metallurgical grade silicon by electron beam melting”, Journal of Materials Processing Technology, vol. 169, no. 1, pp. 16–20, Oct. 2005.
[1]D. Luo, N. Liu, Y. Lu, G. Zhang, and T. Li, “Removal of impurities from metallurgical grade silicon by electron beam melting”, Journal of Semiconductors, vol. 32, no. 3, p. 033003, Mar. 2011.
[1]A. Davis, J. Kennedy, D. Strong, D. Kovalchuk, S. Porter, and P. Prangnell, “Tailoring equiaxed β-grain structures in Ti-6Al-4V coaxial electron beam wire additive manufacturing”, Materialia, vol. 20, p. 101202, Dec. 2021.
[1]J. Etcheverry, N. Mingolo, J. Rocca, and O. Martinez, “A simple model of a glow discharge electron beam for materials processing”, IEEE Transactions on Plasma Science, vol. 25, no. 3, pp. 427–432, Jun. 1997.
[1]И. В. Мельник, “Численное моделирование распределения электрического поля и траекторий частиц в источниках электронов на основе высоковольтного тлеющего разряда”, Известия высших учебных заведений. Радиоэлектроника, vol. 48, no. 6, pp. 61–71, Jun. 2005.
[1]S. Denbnovetsky, J. Felba, V. Melnik, and I. Melnik, “Model of beam formation in a glow discharge electron gun with a cold cathode”, Applied Surface Science, vol. 111, pp. 288–294, Feb. 1997.
J. D. Lawson, The Physics of Charged-Particle Beams. Oxford: Clarendon Press, 1977.
M. Reiser, Theory and Design of Charged Particle Beams. Wiley, 2008, uri: https://www.wiley.com/en-us/Theory+and+Design+of+Charged+Particle+Beams-p-9783527617630. DOI: 10.1002/9783527622047
M. Szilagyi, Electron and Ion Optics. Springer, 2011.
S. Humphries, Charged Particle Beams. New York: Wiley-Interscience, 1990, uri: https://library.uoh.edu.iq/admin/ebooks/76728-charged-particle-beams---s.-humphries.pdf.
B. M. Smirnov, Theory of Gas Discharge Plasma. Springer, 2015, uri: https://www.amazon.com/Theory-Discharge-Springer-Optical-Physics/dp/3319110640. DOI 10.1007/978-3-319-11065-3
M. A. Lieberman, A. J. Lichtenberg, Principles of Plasma Discharges and Materials Processing. Wiley, 2005, uri: https://www.wiley.com/en-us/Principles+of+Plasma+Discharges+and+Materials+Processing%2C+2nd+Edition-p-9780471720010. DOI 10.1002/0471724254
Y. P. Raizer, Gas Discharge Physics. Berlin: Springer-Verlag, 1991. DOI 10.1007/978-3-642-61247-3
[1]И. В. Мельник, “Моделирование энергетической эффективности триодных источников электронов высоковольтного тлеющего разряда с учетом температуры электронов и их подвижности в анодной плазме”, Известия высших учебных заведений. Радиоэлектроника, vol. 60, no. 7, pp. 413–424, Jul. 2017.
[1]I. Melnyk, S. Tuhai, and A. Pochynok, “Interpolation of the Boundary Trajectories of Electron Beams by the Roots from Polynomic Functions of Corresponded Order”, in 2020 IEEE 40th International Conference on Electronics and Nanotechnology (ELNANO), Kyiv, Ukraine, 2020, pp. 28–33.
[1]I. Melnyk, S. Tuhai, and A. Pochynok, “Interpolation Functions for Describing the Boundary Trajectories of Electron Beams Propagated in Ionised Gas”, in 2020 IEEE 15th International Conference on Advanced Trends in Radioelectronics, Telecommunications and Computer Engineering (TCSET), Lviv-Slavske, Ukraine, 2020, pp. 79–83.
[1]I. Melnyk, S. Tuhai, and A. Pochynok, “Calculation of Focal Paramters of Electron Beam Formed in Soft Vacuum at the Plane which Sloped to Beam Axis”, in 2019 International Conference on Information and Telecommunication Technologies and Radio Electronics (UkrMiCo), Odessa, Ukraine, 2019, pp. 1–5.
І. В. Мельник, А. В. Починок, “Дослідження класу алгебричних функцій для інтерполяції межових траєкторій короткофокусних електронних пучків,” Системні дослідження та інформаційні технології, no. 3, pp. 23–39, 2020, doi: https://doi.org/10.20535/SRIT.2308-8993.2020.3.02.
[1]A. F. Tseluyko, V. T. Lazurik, D. L. Ryabchikov, V. I. Maslov, and I. N. Sereda, “Experimental study of radiation in the wavelength range 12.2–15.8 nm from a pulsed high-current plasma diode”, Plasma Physics Reports, vol. 34, no. 11, pp. 963–968, Nov. 2008.
[1]V. Rudychev, V. Lazurik, and Y. Rudychev, “Influence of the electron beams incidence angles on the depth-dose distribution of the irradiated object”, Radiation Physics and Chemistry, vol. 186, p. 109527, Sep. 2021.
[1]V. Lazurik, V. Lazurik, G. Popov, and Z. Zimek, “Two-parametric model of electron beam in computational dosimetry for radiation processing”, Radiation Physics and Chemistry, vol. 124, pp. 230–234, Jul. 2016.
K. D. Mathews, J. H. Fink, Numerical Methods Using MATLAB. Prentice Hall, 1998, uri: https://www.amazon.com/Numerical-Methods-Using-MATLAB-3rd/dp/0132700425/.
И. Н. Бронштейн, К. А. Семендяев, Справочник По Математике Для Инженеров и Учащихся Втузов, 13th ed. Москва: Наука, Гл. ред. физ.-мат. лит., 1986.
J. F. Epperson, An Introduction to Numerical Methods and Analysis. Wiley-Interscience, 2007.
E. Wentzel, L. Ovcharov, Applied Problems of Probability Theory. Moscow: Mir Publishers, 1986, uri: https://mirtitles.org/2022/06/03/applied-problems-in-probability-theory-wentzel-ovcharov/.
N. R. Draper, H. Smith, Applied Regression Analysis. Wiley, 1998, uri: https://www.wiley.com/en-us/Applied+Regression+Analysis,+3rd+Edition-p-9780471170822. DOI 10.1002/9781118625590
J. A. Gubner, Probability and Random Processes for Electrical and Computer Engineers. Cambridge, UK: Cambridge University Press, 2006. DOI 10.1017/CBO9780511813610
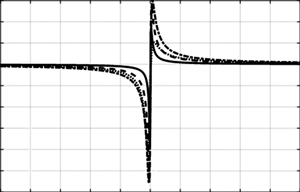
##submission.downloads##
Опубліковано
Як цитувати
Номер
Розділ
Ліцензія
Авторське право (c) 2023 Вісті вищих учбових закладів. РадіоелектронікаИздатель журнала Известия высших учебных заведений. Радиоэлектроника (сокр. "Известия вузов. Радиоэлектроника"), Национальный технический университет Украины "Киевский политехнический институт", учитывает, что доступ автора к его статье является важным как для самого автора, так и для спонсоров его исследований. Мы представлены в базе издателей SHERPA/RoMEO как зеленый издатель (green publisher), что позволяет автору выполнять самоархивирование своей статьи. Однако важно, чтобы каждая из сторон четко понимала свои права. Просьба более детально ознакомиться с Политикой самоархивирования нашего журнала.
Политика оплаченного открытого доступа POA (paid open access), принятая в журнале, позволяет автору выполнить все необходимые требования по открытому доступу к своей статье, которые выдвигаются институтом, правительством или фондом при выделении финансирования. Просьба более детально ознакомиться с политикой оплаченного открытого доступа нашего журнала (см. отдельно).
Варианты доступа к статье:
1. Статья в открытом доступе POA (paid open access)
В этом случае права автора определяются лицензией CC BY (Creative Commons Attribution).
2. Статья с последующим доступом по подписке
В этом случае права автора определяются авторским договором, приведенным далее.
- Автор (каждый соавтор) уступает Издателю журнала «Известия высших учебных заведений. Радиоэлектроника» НТУУ «КПИ» на срок действия авторского права эксклюзивные права на материалы статьи, в том числе право на публикацию данной статьи издательством Аллертон Пресс, США (Allerton Press) на английском языке в журнале «Radioelectronics and Communications Systems». Передача авторского права охватывает исключительное право на воспроизведение и распространение статьи, включая оттиски, переводы, фото воспроизведения, микроформы, электронные формы (он- и оффлайн), или любые иные подобные формы воспроизведения, а также право издателя на сублицензирование третьим лицам по своему усмотрению без дополнительных консультаций с автором. При этом журнал придерживается Политики конфиденциальности.
- Передача прав включает право на обработку формы представления материалов с помощью компьютерных программам и систем (баз данных) для их использования и воспроизводства, публикации и распространения в электронном формате и внедрения в системы поиска (базы данных).
- Воспроизведение, размещение, передача или иное распространение или использование материалов, содержащихся в статье должно сопровождаться ссылкой на Журнал и упоминанием Издателя, а именно: название статьи, имя автора (соавторов), название журнала, номер тома, номер выпуска, копирайт авторов и издателя "© Национальный технический университет Украины "Киевский политехнический институт"; © автор(ы)".
- Автор (каждый соавтор) материалов сохраняет все права собственника материалов, включая патентные права на любые процессы, способы или методы и др., а также права на товарные знаки.
- Издатель разрешает автору (каждому соавтору) материалов следующее:
- Право пользоваться печатными или электронными вариантами материалов статьи в форме и содержании, принятыми Издателем для публикации в Журнале. Подробнее см. политики Оплаченного открытого доступа, подписки и самоархивирования.
- Право бесплатно копировать или передавать коллегам копию напечатанной статьи целиком или частично для их личного или профессионального использования, для продвижения академических или научных исследований или для учебного процесса или других информационных целей, не связанных с коммерческими целями.
- Право использовать материалы из опубликованной статьи в написанной автором (соавторами) книге, монографии, учебнике, учебном пособии и других научных и научно-популярных изданиях.
- Право использовать отдельные рисунки или таблицы и отрывки текста из материалов в собственных целях обучения или для включения их в другую работу, которая печатается (в печатном или электронном формате) третьей стороной, или для представления в электронном формате во внутренние компьютерные сети или на внешние сайты автора (соавторов).
- Автор (соавторы) соглашаются, что каждая копия материалов или любая ее часть, распространенная или размещенная ими в печатном или электронном формате, будет содержать указание на авторское право, предусмотренное в Журнале и полную ссылку на Журнал Издателя.
- Автор (соавторы) гарантирует, что материалы являются оригинальной работой и представлены впервые на рассмотрение только в этом Журнале и ранее не публиковались. Если материалы написаны совместно с соавторами, автор гарантирует, что проинформировал их относительно условий публикации материалов и получил их подписи или письменное разрешение подписываться от их имени.
- Если в материалы включаются отрывки из работ или имеются указания на работы, которые охраняются авторским правом и принадлежат третьей стороне, то автору необходимо получить разрешение владельца авторских прав на использование таких материалов в первом случае и сделать ссылку на первоисточник во втором.
- Автор гарантирует, что материалы не содержат клеветнических высказываний и не посягают на права (включая без ограничений авторское право, права на патент или торговую марку) других лиц и не содержат материалы или инструкции, которые могут причинить вред или ущерб третьим лицам. Автор (каждый соавтор) гарантирует, что их публикация не приведет к разглашению секретных или конфиденциальных сведений (включая государственную тайну). Подтверждением этого является Экспертное заключение (см. перечень документов в Правила для авторов).
- Издатель обязуется опубликовать материалы в случае получения статьей положительного решения редколлегии о публикации на основании внешнего рецензирования (см. Политика рецензирования).
- В случае публикации статьи на английском языке в журнале «Radioelectronics and Communications Systems» (Издатель: Аллертон Пресс, США, распространитель Springer) автору (соавторам) выплачивается гонорар после выхода последнего номера журнала года, в котором опубликована данная статья.
- Документ Согласие на публикацию, который подают русскоязычные авторы при подаче статьи в редакцию, является краткой формой данного договора, в котором изложены все ключевые моменты настоящего договора и наличие которого подтверждает согласие автора (соавторов) с ним. Аналогичным документом для англоязычных авторов является Copyright Transfer Agreement (CTA), предоставляемый издательством Allerton Press.
- Настоящий Договор вступает в силу в момент принятия статьи к публикации. Если материалы не принимаются к публикации или до публикации в журнале автор (авторы) отозвал работу, настоящий Договор не приобретает (теряет) силу.